HSC1102 – Radiation Physics & Dosimetry – Atomic Physics
- YouWei
- Mar 22, 2018
- 4 min read

Here’s a basic outline of this chapter:
Atomic Model
Dalton
Thomson
Rutherford
Bohr
Schrödinger
Isotopes, Isotones, Isobars, Isomers
Energy Band Theory
X-Ray Notation
Excitation / Ionisation
Binding Energy
The Ångstrom
Electron Volts (eV)
Atomic Number (Z) and Atomic Mass (A)
History of the Atomic Model
There are 5 main models we will explore, each built on the previous one, and each adding defining features to the model, eventually making it the model in use today.
Dalton’s Greek Model (1803) – Atoms are small, indivisible objects
Thomson’s Plum Pudding Model (1904) – Atoms constitute free floating positive and negative charges
Rutherford’s Nuclear Model (1911) – A positively charged nucleus sits in a negatively charged cloud
Bohr’s Electron Shell Model (1913) – A positively charged nucleus is orbited by negatively charged electrons in neat shells
Schrodinger’s Electron Cloud Model (1926) – Electrons are described as probabilistic wave functions and exist within close proximity to the nucleus
The three fundamental particles that exist within an atom, otherwise known as subatomic particles, are the proton, the neutron and the electron.
While the proton and neutron have relatively equal mass and reside within the central nucleus, electrons are 1836 times lighter and exist within close proximity to the nucleus.
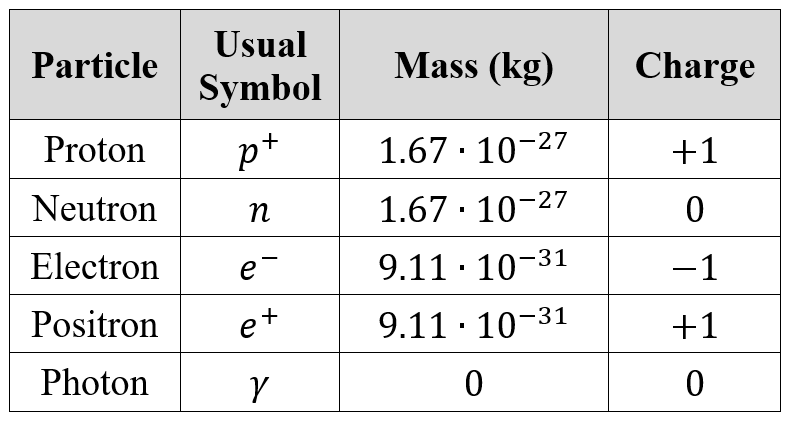
The table above shows the properties of each subatomic particle, and also introduces a couple of new, unmentioned ones.
The positron is the antiparticle counterpart of the electron. It is akin to an electron, with similar mass, just with an opposite charge. Some forms of radioactive decay will generate positrons.
A photon is a packet of energy. It is massless, neutral in charge (no charge) and travels at the speed of light. Any radiation, ionising or non-ionising, is carried through a photon. Visible light is made up of photons, likewise X-Rays, gamma rays and even microwaves.
The speed of light is usually denoted with the symbol c.

The identity (element) of an atom depends on its atomic number. This is also the number of protons within the nucleus of the atom.
For example, a chlorine atom will always have 17 protons in its nucleus, and an oxygen atom will always have 8 protons in its nucleus.
The nucleon number, or atomic mass, is the total number of particles in the nucleus. This is obtained by adding together the total number of protons and neutrons within the nucleus, which may not be an equal number. A nucleon is any particle that belongs to the nucleus.
On a periodic table, we see each element with their atomic mass on the top, and atomic number towards the bottom left:
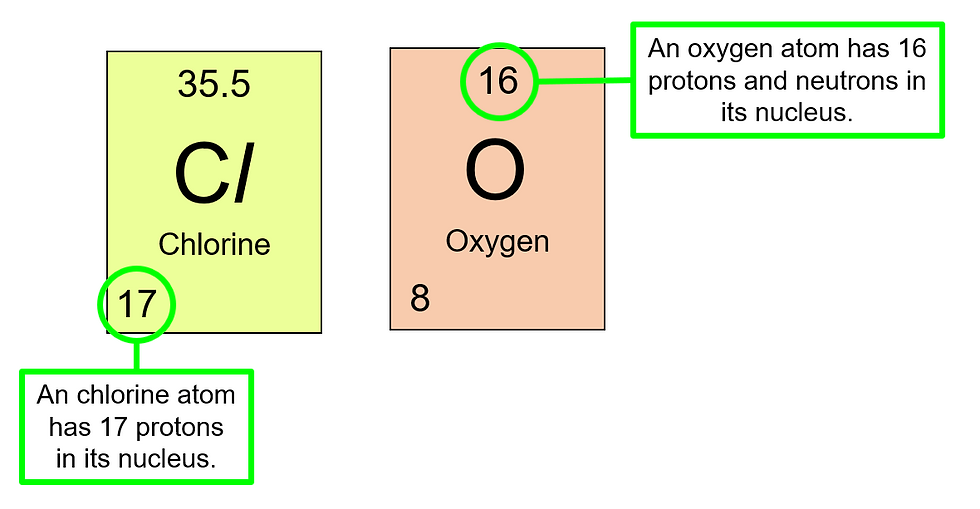
Notice that the number of neutrons does not depend on the number of protons.
Isotopes are atoms of the same element (which means they have the same atomic number) but different nucleon numbers (which means they have a different number of neutrons).
An example is Cl-35 and Cl-37. The atomic mass or nucleon number is written after a dash to the right of the chemical symbol. Since only the mass number and atomic number are provided, the number of neutrons has to be calculated.
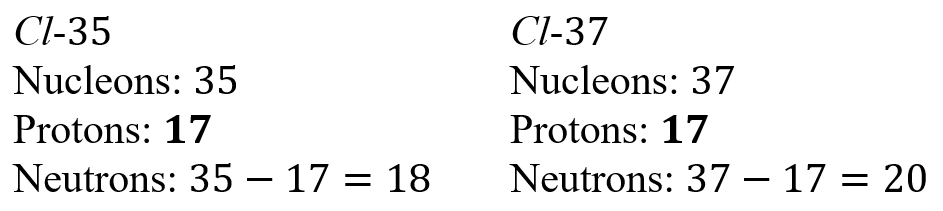
Isotones are atoms with the same number of neutrons, but different number of protons (different identity).
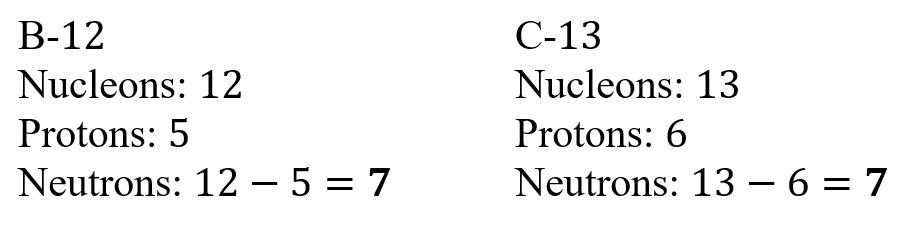
Isobars are atoms with the same atomic mass, which means they have the same number of nucleons.

A nuclear isomer is one with the same composition of the nucleus, but it exists at a different energy state. As such, the nucleus is made up of the same number of protons and neutrons, but the energy levels are different, and the one with a higher energy state can decay into the lower energy state one by emitting gamma rays.
An example of this is Tc-99m and Tc-99. The m behind the atomic mass stands for metastable, and signifies that this atom can decay into the other.
Energy Band Theory
The electrons are organized into different shells each with a distinct energy level, and starting from the innermost shell closest to the nucleus, the shells are named K, L, M, N, O, and so on.
The binding energy of an electron is the energy that the electron must receive before it has the ability to break free from the influence of the nucleus. The closer the electron is to the nucleus, the harder it is to separate from the nucleus, and hence the larger the binding energy.
Non-ionising radiation are low energy electromagnetic (EM) waves, and they constitute the near-UV and lower. On the contrary, ionizing radiation creates ions when its photons interact with matter.
Shells are usually depicted like this:

The energy levels are in negative values it represents an attractive force (the electron is attracted to the nucleus), and it symbolises an energy “deficit” that the electron has to overcome (gain) in order to break free from the influence of the nucleus.
In short, any electron in the shell can be viewed as having a net negative energy, and any energy gain can let it reach 0, where it is a free electron, free from the influence of the nucleus.
Excitations, or ionisations, are caused by photon interaction, or collisions with other particles. They can also be caused by sufficient heat. The principle is that the electrons will gain enough energy to overcome its binding energy, and the atom will lose an electron through excitation, leaving behind a positively charged ion. Any surplus energy that was absorbed by the electron will contribute to the outgoing electron’s kinetic energy.
In the event that an electron has to move from one shell to another, the energy surplus (surplus because typically electrons move down the shells, closer to the nucleus) can be calculated as the difference in binding energy between the two shells.
Hence an electron transitioning from the L shell to the K shell for this atom will liberate this amount of energy in the form of a photon:

Some new units that are introduced to us include the Ångstrom and the Electron-Volt (eV).
The Ångstrom is a unit measuring length.
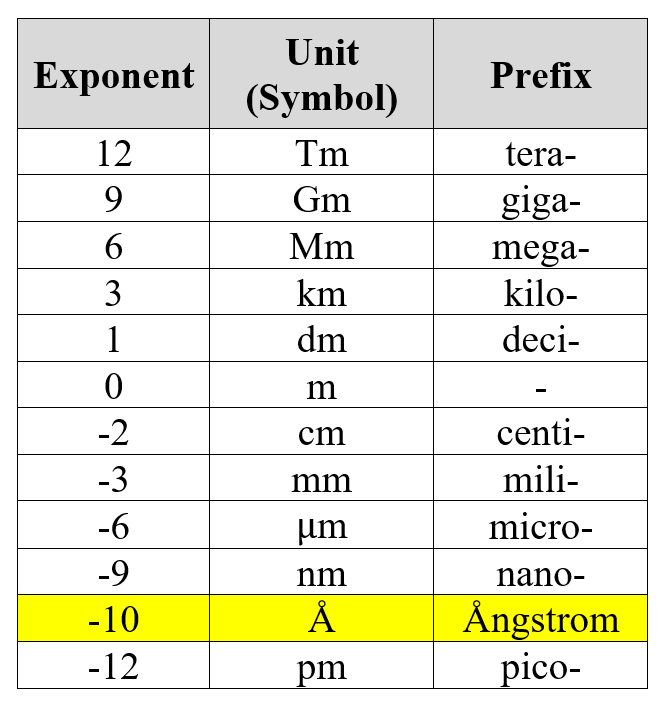
The common units of lengths are where the exponents are multiples of 3, and the Ångstrom is one of the non-standard units that happens to be quite widely used in this area of Physics.
The electron-Volt is a measure of energy. One electron-Volt is the amount of energy gained (or lost) by a single electron moving through an electric potential difference of one volt.

Typically, the energy level of diagnostic X-Rays are in the keV range, while therapeutic X-Rays are in the MeV range.
Comments